Organisms with multiple sets of chromosomes must balance their gene expression levels to survive. By analyzing dominance patterns and chromatin accessibility in strawberries, Fang et al. described the mechanism of subgenome dominance that allows strawberries and other allopolyploid species to survive.
This webpage was produced as an assignment for an undergraduate course at Davidson College.
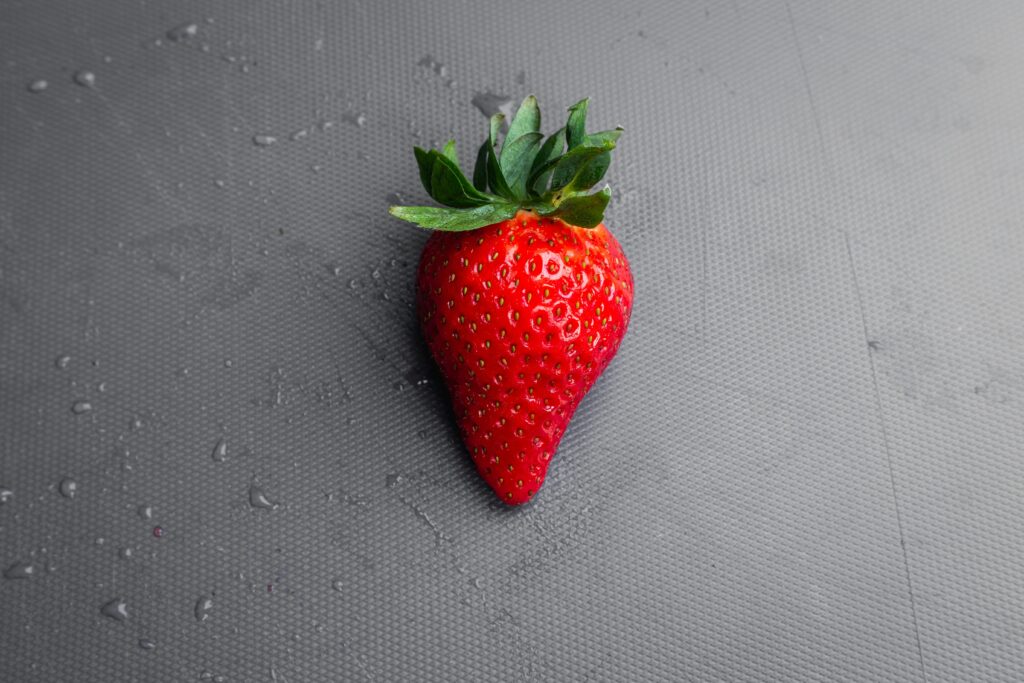
About the author: Nicolas Pinzon. For further information please get in touch with Nico Pinzon at nipinzon@davidson.edu
Weird things happen in nature. Understanding how these happen helps us push the boundaries of our biological understanding. Repeatedly throughout history, we have adapted these weird nature-given mechanisms for therapeutic and research use, solving problems that once seemed unsolvable with methods once unthinkable.
Allopolyploid species have two or more entire sets of chromosomes. Regulating how much of each gene is expressed is essential for the survival of such species, as too many copies of the same gene can result in downstream detrimental effects1. To overcome this, allopolyploid species have evolved mechanisms to turn certain chromosomes off, often resulting in a dominant subgenome2. But, although we know that this happens, debate over the exact mechanism that makes subgenome dominance possible remains. Writing in Nature Communications, Fang et al.3 describe the mechanism behind subgenome dominance in octoploid strawberries, accurately expanding on the Mendelian genetic principles that guide our research and therapeutic efforts and are commonly taught in schools. Oh, and yes that is right, the same cultivated strawberries we eat daily.
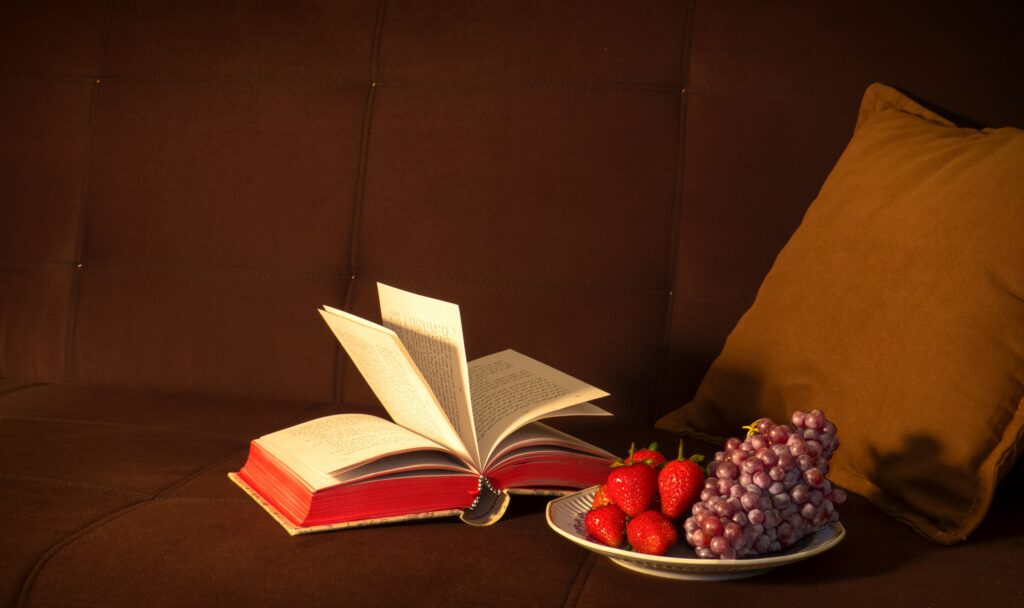
For a gene to be transcribed, loads of molecular machinery must bind either directly to the gene or to the non-coding regions before it. Think of someone trying to grip one of your middle fingers with one of their hands. If you had your first closed, then it would be impossible, or at least very hard, for them to access it. Instead, if you had your hand wide open, they could go right in and grip it. Similarly, for a gene to be transcribed, the DNA region in which the gene is located must be easily accessible. Researchers of the Fang et al. group hypothesized that differences and constant changes in the accessibility of genomes could be the reason strawberries and other allopolyploid species exhibit a dominant subgenome.
To validate this hypothesis, the group started by making a map of easily accessible regions in the subgenomes of the strawberry. They used a combination of enzymes that bind and degrade accessible DNA and genomic sequencing to develop this map. Their results showed that subgenome A, the dominant subgenome in strawberries, had significantly more easily accessible regions than subgenomes B, C, and D. Furthermore, these easily accessible regions were significantly longer in subgenome A than the rest. Next, researchers turned to regions that preceded genes in each subgenome (because these preceding regions often have regulatory elements binding to them that drive gene expression). They found that in subgenome A, preceding regions were widely more accessible than in subgenomes B, C, and D. Together, these results make the case that DNA accessibility is highly correlated with and could be driving subgenome dominance in strawberries and other allopolyploid species.
But researchers did not stop there. To further clarify the picture of how there are subgenomes that are preferentially expressed over others, researchers in the Fang et al. group turned to analyzing the patterns of genes expressed that are of subgenome A origin vs. of different subgenome origin. They found that 72% of dominant subgenome A genes were expressed preferentially over their homologs (equivalent genes in different chromosomes) in other subgenomes. Using computational approaches, they discovered that these homologous genes accumulated multiple deleterious mutations in the non-dominant subgenomes. In fact, this was still the case for genes that were preferentially expressed in subgenomes other than subgenome A. If a gene is preferentially expressed in one subgenome, then its homologs will accumulate mutations, including gene fragmentation in some cases, that further drive their expression down. Further, using enzymatic analysis of the DNA accessibility of homologous genes, researchers found that the non-dominant homologs also exhibited lower levels of accessibility.
In conclusion, the Fang et al. group proposes that DNA accessibility drives subgenome dominance in strawberries. Furthermore, they propose a model under which small differences are continuously amplified, as non-dominant homologs accumulate mutations that continuously drive expression and accessibility down. Finally, although the evidence is strong, the authors present one big caveat to their research. Strawberries have constantly undergone selective sweeps associated with their domestication, in efforts to make them bigger, juicier, and more nutritive. This could have caused subgenome A to be driven to dominance artificially. This could mean that in other organisms or under different selective circumstances, subgenome dominance could not be exacerbated naturally. Rather, the deleterious mutations we observe are a factor of human selective forces. Performing subsequent experimentation with strawberries of different ancestry and with other plant species is warranted.
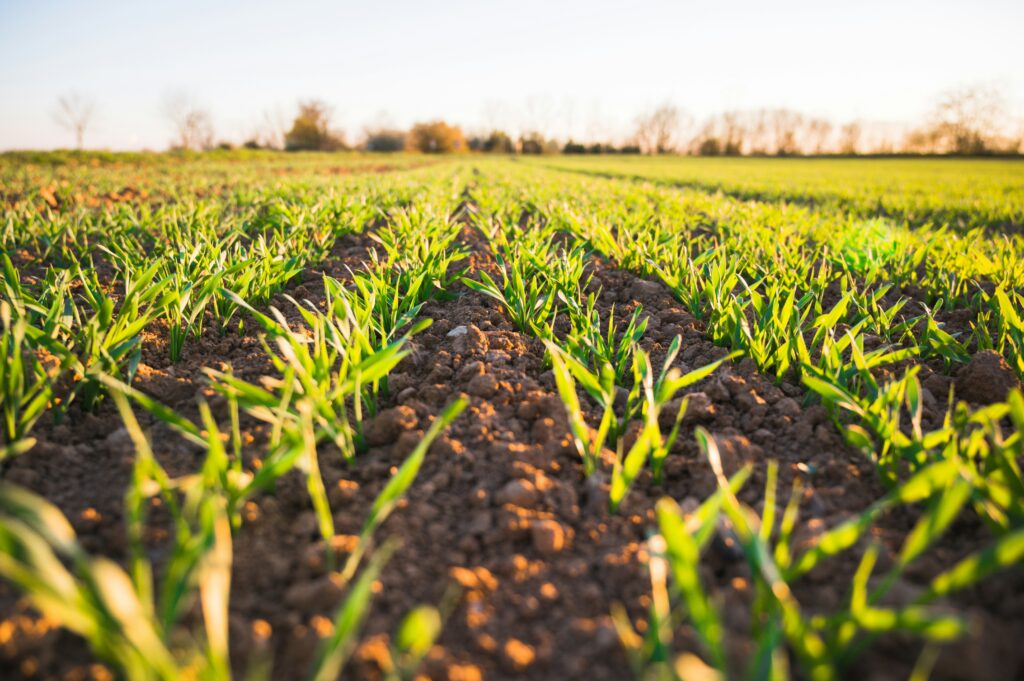
Nevertheless, understanding how subgenome dominance is driven has enormous agricultural implications. Wild strawberries look very different than you think. They are extremely small and have lots of pointy protrusions. You probably would not want to eat them if you found them in the wild. Yet, by artificial mating and constant selection, we have harnessed octoploid strawberries that are ~10x bigger than their wild counterparts, and much more delicious. Without knowing, we drove subgenome A to dominance in these octoploid strawberries. This same process could be applied to other common fruits and vegetables. Further, with today’s technology and knowing that DNA accessibility drives subgenome dominance, we could biochemically target genomic regions of interest and drive them to dominance by making them easily accessible. Such applications can improve our crop yield under harsh conditions, reduce economic costs, and amplify agricultural variety.
However, before we see such a wide array of benefits, a big ethical dilemma remains unsettled. Should we freely allow the genetic manipulation of allopolyploids, which most of our most common crops happen to be, in agriculture? Driving subgenome dominance, whether it is done artificially or naturally, continuously brings genetic diversity down. In nature, this is a big deal. Low genetic diversity makes organisms more prone to extinction4. Further, the accumulation of deleterious mutation aggravates this by significantly reducing organismal fitness5. For both the species themselves and those who, like us, feed on these crops, this is not good. Additionally, such genetic influence could be taken advantage of by big companies with the primary goal of making more money. Driving subgenome dominance with the wrong goals could result in negative health effects for consumers and could lead to strains of crops that may become invasive and uncontrollable.
All in all, elucidating the molecular mechanisms behind the weird things that happen in nature holds great potential for good, but regulations must be established so that we can prevent misapplications of such principles.
References
© Copyright 2022, Department of Biology, Davidson College, Davidson, NC 28036.
Nico, I think this is a great summary of this paper! I especially like how you break each concept covered down into easily understandable metaphors, like with the finger versus a fist and DNA polymerase binding. Though I knew that many plants had multiple copies of their genome, I didn’t know much about how this worked in practice and the presence of subgenomes. I wonder about the possibilities of these subgenomes being artificially made more dominant in strawberries and how wild plants may survive with these multiple copies of genes potentially having more expression. I also like your evaluation of the conservational and ethical drawbacks of this kind of genetic modification in agriculture – monoculture and sterile crops are definitely a big issue which we may see become an even greater one in the near future. Overall great article.
P.S. Wild strawberries actually taste great!
This was a super fascinating article! I don’t get to read a lot of plant-related papers, so the whole idea of allopolyploids and subgenomes is a new topic! I appreciated your analogies and explanations! I would love to learn more about the different subgenomes of the strawberry and how they compare to each other.
I loved your discussion of the ethical implications, because I think it’s a super important topic in agriculture. Just because we CAN do something, doesn’t mean we SHOULD, and I think people are becoming more comfortable with grappling and discussing this idea in science. This makes me think of our common banana, which is a cross between two different plants. Because of this, bananas are triploid, and so cannot reproduce on their own. This is a huge downside to our manipulation in natural goods, and I think it’s important to consider these consequences in this type of research!