Disclaimer: This web page was produced as an assignment for an undergraduate course at Davidson College.
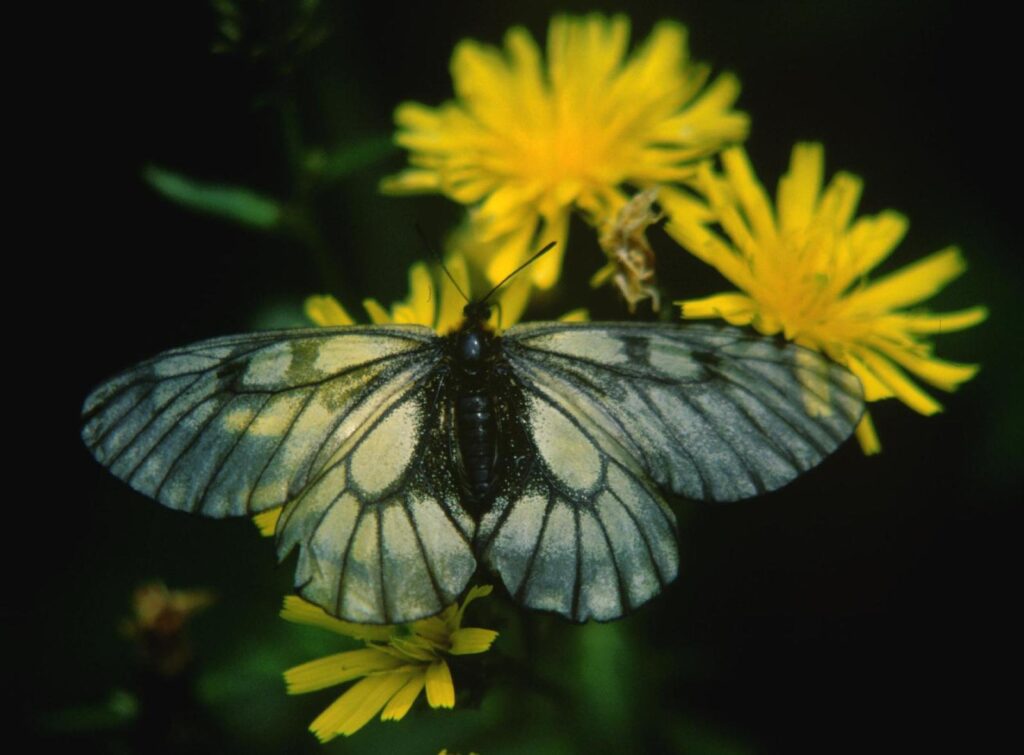
Genome sequencing of Parnassius glacialis reveals broad expansion and selective evolution, with genetic differences between high and low-altitude populations indicating past bottlenecks caused increased adaptation to lower altitudes.
As our world undergoes constant change, so too do our ecosystems, prompting evolution and adaptation. Throughout history, various events, from ice ages to climate shifts, have altered environments and as a result shaped life’s diversity. Parnassius glacialis, the Glacial Apollo butterfly, is typically found at high altitudes in the Qinghai-Tibet plateau and has experienced significant change. Recent uplift and habitat fragmentation have led to speciation that has divided the species across altitudes and resulted in clear differences between high-altitude and low-altitude butterflies.1 While we know these changes are happening, it is not easy to determine what is controlling the evolution and adaptation in Parnassius glacialis, as we have been able to do with other organisms. This is due to the lack of genomic information on this species. Part of the interest in sequencing the genome of this alpine butterfly was to pinpoint the underlying processes behind its genomic evolution to better how speciation occurred, which is exactly what Zhao et al 1 did.
Population isolation, a result of geographic barriers, plays a crucial role in the evolution of species like P. glacialis. This isolation, often caused by features like mountains or rivers, prevents gene flow between isolated populations and the rest of the species, leading to genetic divergence over time.2 As the isolated population adapts to its unique environment, it may eventually become distinct enough to be considered a separate species. Parnassius itself arose during the Late Eocene to middle Miocene era almost 16.99 million years ago, but divergences due to the uplift of the Qinghai-Tibet Plateau (QTP) did not occur until 9.69-4.12 million years ago. Even then, it was not until ~0.95 million years ago that P. glacialis emerged, following the Kunlun-Huanghe tectonic movement.3 Diversification rates between species usually increase in concordance with physical changes in an environment.5 Due to the many divergences over several million years, the organisms that make up the genus Parnassius gradually developed a larger genome compared to other butterflies of the family Papilionidae.4 This shows how geographic barriers or events cause dispersal that ultimately ends up in species diversification.1,2,4
The researchers first observed six different Parnassius species occupying different altitudes, including 41 P. glacialis butterflies from nine different populations at varying altitudes across the Qinghai-Tibet Plateau. In doing this, they wanted to look at the effects of transposable elements (TEs) in relation to the species’ large genome to assess differentiation between populations at different altitudes. Genome sizes were gathered from the six different species of Parnassius butterflies, ranging from 1.0-1.40 Gigabases (a measurement used to understand length of DNA. One gigabase is equivalent to one billion bases) respectively, with species at lower altitudes having much larger genome sizes. The genome size for P. glacialis was estimated to be 1.33-1.35 Gb, one of the largest. Through annotating its coding genes at 1.35 Gb, researchers found that transposable elements made up a significant 68% of the genome, indicating rapid accumulation of transposable elements.
Furthermore, the researchers performed a gene family analysis and identified 703 expanded orthologous genes in P. glacialis, indicating genes that have arisen from speciation events. Remember that these events can be rapid or abrupt which usually impact clade-lineages, which are groupings of living and extant organisms that share a common ancestor.5 These expanded groups were enriched in ribosome signaling pathways, particularly the acidic ribosomal P protein gene family (RPLP), which supports processes like protein synthesis and anti-oxidation, crucial for organism function.6 434 of these genes were widely distributed across the genome of P. glacialis, with most having pseudogene properties. Pseudogenes structurally resemble genes but cannot encode proteins, and in P. glacialis specifically, they evolved faster than in any other species, possibly aiding in adaptation outside the Qinghai-Tibet Plateau.
To directly observe the relationship between different samples of P. glacialis across the Qinghai-Tibet Plateau, researchers also performed a comparative analysis. It demonstrated lower genetic diversity in populations at lower altitudes than in those at higher altitudes. This could be due to environmental stressors such as increased predation and hotter temperatures, as populations spread out across the plateau and faced more climate change following habitat damage from uplift. These stressors likely contributed to the higher number of transposable elements in lower-altitude populations which can suggest that the species likely dispersed during a colder period and suffered a bottleneck after the last glacial maximum, possibly due to insufficient adaptation to the low-altitude environment.
So, now that we understand how this divergence occurred, what implications does it hold for future studies? The sequenced genome of P. glacialis offers a robust framework of genomic information, not only shedding light on the biological changes of organisms from the Qinghai-Tibet Plateau but also providing insights into ecosystems worldwide. However, ethical considerations loom large. Given the low genetic diversity of the low-altitude butterflies that comprise this species, it’s crucial to handle them with care. The collection of these organisms for scientific purposes can inadvertently increase their risk of extinction. Without careful management, this diverged butterfly species could vanish, depriving us of valuable insights for future studies. In future studies, this data can help us infer the processes of speciation and divergence for other alpine butterflies and anticipate their future evolution as our world continues to change. Nevertheless, we must approach each scenario involving different organisms in an ethical and delicate matter.
References:
- Zhao., Y., Su, C., He., B., Nie, R., Wang, Y., Ma, J., Song, J., Yang, Q., & Hao, J. Dispersal from the Qinghai-Tibet plateau by a high-altitude butterfly is associated with rapid expansion and reorganization of its genome. Nature communications, 14(1), 8190 (2023). https://doi.org/10.1038/s41467-023-44023-2
- Tao, R., Xu, C., Wang, Y., Sun, X., Li, C., Ma, J., Hao, J., & Yang, Q. Spatiotemporal Differentiation of Alpine Butterfly Parnassius glacialis (Papilionidae: Parnassiinae) in China: Evidence from Mitochondrial DNA and Nuclear Single Nucleotide Polymorphisms. Genes, 11, 188 (2020). https://doi.org/10.3390/genes11020188
- Zhao., Y. He., B. Tao., R. Su., C., Ma, J., Hao, J., Yang, Q. Phylogeny and Biogeographic History of Parnassius Butterflies (Papilionidae: Parnassiinae) Reveal Their Origin and Deep Diversification in West China. Insects 13, 406 (2022) https://doi.org/10.3390/insects13050406
- Li, X., Fan, D., Zhang, W. et al. Outbred genome sequencing and CRISPR/Cas9 gene editing in butterflies. Nat Commun 6, 8212 (2015). https://doi.org/10.1038/ncomms9212
- Condamine, F. L., Rolland, J., Höhna, S., Sperling, F. A. H., & Sanmartín, I. Testing the Role of the Red Queen and Court Jester as Drivers of the Macroevolution of Apollo Butterflies. Systematic biology, 67(6), 940–964 (2018). https://doi.org/10.1093/sysbio/syy009
- Hoerter JE and Ellis SR. Biochemistry, Protein Synthesis. In: StatPearls [Internet]. Treasure Island (FL): StatPearls Publishing; (2024). https://www.ncbi.nlm.nih.gov/books/NBK545161/
- Zhang, Y. A hierarchical analysis of ecosystem classification with implementing in two continental ecoregions. Environ Syst Res 10, 39 (2021). https://doi.org/10.1186/s40068-021-00243-3
For any questions related to this post, please reach out to Abraham Hernandez Mujica (abhernandezmujica@davidson.edu)
© Copyright 2024 Department of Biology, Davidson College, Davidson, NC 28036
I think research like this is so important for conservation efforts, especially with climate change having such a profound effect today. By studying the species at different altitudes, there could be better insights into how their genes are changing in response to the environment. It’s interesting that genes arose specifically from speciation events because that may relate so strongly to the environment as barriers prevent continued reproduction. I think this type of research should be expanded into other species to prevent the die out of so many amazing species and genetic diversity in order to maintain life on Earth.
This is a well written and very thorough evaluation of your scientific article, and a cool topic of choice too! I think I’d like to know more about some of the concepts mentioned here, like ribosome signaling pathways and ‘pseudogenes’, I only know a basic amount about the first and have never heard of the second, so I think it’d be cool to understand how these things may relate to increased chance of survival. I think you make a good point about why it’s important to continue these studies as climates are changing and these butterflies seem to be a very delicate species, though I wonder how genomics might be able to help conservation efforts outside of the conservation of genetic data.